Material Use Guide for Metal Additive Manufacturing
In this guide, we’ll provide an in-depth understanding of metal materials commonly used in metal additive manufacturing, along with selection criteria and factors to consider during the material selection process, material properties, and industry-specific applications. The information presented in this guide will help you make informed decisions when choosing materials for your metal AM projects.
Metal Additive Manufacturing: Choosing Materials with Purpose
Metal additive manufacturing (MAM) encompasses a collection of innovative technologies that fabricate parts through the precise, layer-by-layer deposition of material based on a digital model. MAM has revolutionized the manufacturing landscape, enabling unparalleled design flexibility, reduced material waste, and the capacity to generate intricate geometries that were previously unattainable or cost-prohibitive with conventional manufacturing techniques.
Material selection plays a critical role in the success of metal AM projects. The choice of material affects the mechanical, thermal, and chemical performance of the final part and influences the AM process. Selecting the appropriate material can optimize part performance, minimize post-processing requirements, and reduce overall production costs.
Material Selection Criteria for Metal Additive Manufacturing
Selecting the appropriate metal material is a crucial step in the additive manufacturing process, as it directly impacts the performance, quality, and cost-effectiveness of the final part. With a wide range of materials available, understanding the key selection criteria is essential to making informed decisions that align with the intended application and specific requirements.
In this section, we’ll discuss the fundamental factors to consider when selecting materials for metal additive manufacturing, including:
- Mechanical Properties
- Thermal Properties
- Chemical Resistance & Corrosion Behavior
- Surface Finish & Detail Resolution
- Cost & Availability
- Post-Processing Requirements
By evaluating these criteria, you can optimize your material selection process, ensuring the success of your metal additive manufacturing projects.
Mechanical Properties |
Thermal Properties |
The mechanical properties of a material, such as tensile strength, fatigue resistance, and ductility, are critical factors to consider when selecting materials for metal AM. The chosen material should meet or exceed the requirements of the intended application in terms of load-bearing capacity, durability, and resistance to deformation or failure. | Thermal properties, including thermal conductivity, specific heat capacity, and coefficient of thermal expansion, are important factors to consider for parts that will be exposed to varying temperatures or thermal loads. Selecting materials with suitable thermal properties can help mitigate issues such as warping, thermal stress, or premature failure. |
Chemical Resistance & Corrosion Behavior |
Surface Finish & Detail Resolution |
For parts that will be exposed to corrosive environments, chemical resistance, and corrosion behavior are crucial factors to consider. Material selection should account for the specific chemical and environmental conditions the part will encounter in service, ensuring long-term reliability and performance. | The surface finish and level of detail achievable with a specific material are important factors to consider, especially for parts with complex geometries or intricate features. Material properties such as particle size and melting behavior can impact the resolution and surface quality of the final print. |
Cost & Availability |
Post-Processing Requirements |
The cost and availability of materials can significantly impact the overall cost and feasibility of a metal AM project. Factors such as material cost, processing requirements, and post-processing steps should be taken into account when selecting materials. | Some materials may require additional post-processing steps, such as heat treatment, surface finishing, or machining, to achieve the desired part properties and performance. Consideration should be given to the required post-processing steps and their potential impact on the overall production timeline and cost. |
Commonly Used Materials in Additive Manufacturing
The choice of material plays a vital role in the success of any metal AM project, as it directly influences the performance, quality, and cost-effectiveness of the final part. With the rapid development of MAM technologies, an extensive range of metal materials has become available, each exhibiting unique properties and benefits.
The most commonly used metal materials in additive manufacturing include:
- Aluminum Alloys
- Titanium Alloys
- Stainless Steel
- Nickel-Based Superalloys
- Cobalt-Chrome Alloys
- Tool Steels
- Precious Metals
1. Aluminum Alloys
Aluminum alloys are lightweight, corrosion-resistant, and exhibit good strength-to-weight ratios. They have high thermal and electrical conductivity, making them suitable for applications requiring active heat dissipation. Commonly used aluminum alloys in MAM include AlSi10Mg and AlSi12.
2. Titanium Alloys
Titanium alloys are known for their high strength-to-weight ratio, corrosion resistance, and biocompatibility. They have excellent fatigue and fracture resistance, making them suitable for demanding applications. Ti-6Al-4V (Grade 5) is the most common titanium alloy used in MAM.
3. Stainless Steel
Stainless steels are iron-based alloys with a minimum of 10.5% chromium content. They offer excellent corrosion resistance, mechanical strength, and ductility. Common stainless steel grades used in MAM include 316L, 17-4PH, and 15-5PH.
4. Nickel-Based Superalloys
Nickel-based superalloys are characterized by their high strength, corrosion resistance, and excellent high-temperature performance. They maintain their mechanical properties in extreme environments and have good creep resistance. Inconel 718 and Hastelloy X are common nickel-based superalloys used in MAM.
5. Cobalt-Chrome Alloys
Cobalt-chrome alloys are known for their high strength, wear resistance, and biocompatibility. They have excellent corrosion resistance and maintain their mechanical properties at elevated temperatures. CoCrMo and CoCrW alloys are frequently used in MAM.
6. Tool Steels
Tool steels are high-carbon, alloyed steels designed for applications requiring excellent hardness, wear resistance, and toughness. They can be heat-treated to achieve various hardness levels and are suitable for metalworking tools and molds. Maraging steels, such as MS1 and H13, are common tool steels used in MAM.
7. Precious Metals
Precious metals, such as gold, silver, and platinum, are valued for their rarity, corrosion resistance, and aesthetic appeal. They have high ductility, making them suitable for intricate designs and custom applications.
Microstructure & Phase Transformations
In metal additive manufacturing, the microstructure and phase transformations of the material play a critical role in determining the final part’s properties and performance. The MAM process subjects the material to rapid heating and cooling cycles, resulting in unique microstructures and potential phase transformations.
Here, we discuss the key aspects of microstructure and phase transformations in MAM, highlighting their influence on material properties, process parameters, and post-processing techniques.
Microstructure
Microstructure refers to the arrangement of grains, phases, and defects within a material on a microscopic scale. The microstructure in MAM is influenced by factors such as material composition, process parameters (e.g., energy input, layer thickness, and scan strategy), and thermal history.
Microstructure Influence on Material Properties |
The microstructure of a material has a direct impact on its mechanical, thermal, and chemical properties. Different microstructures can result in variations in strength, ductility, fatigue resistance, and corrosion resistance. Understanding and controlling the microstructure in MAM is crucial for optimizing the final part’s properties. |
Phase Transformations
Phase transformations occur when a material undergoes a change in its crystal structure or phase due to changes in temperature, pressure, or composition. Phase transformations include solid-state transformations (e.g., precipitation or dissolution of secondary phases) and solid-liquid transformations (e.g., melting and solidification).
Phase transformations in metal additive manufacturing are influenced by the material composition, process parameters (e.g., energy input, cooling rate, and thermal gradients), and post-processing techniques, such as heat treatment or hot isostatic pressing.
Phase Transformation Influence on Material Properties |
Phase transformations can significantly impact the material properties and performance of the final part. For example, certain phase transformations can result in increased strength or improved corrosion resistance, while others can lead to embrittlement or reduced ductility. |
Controlling Microstructure & Phase Transformations
Optimizing process parameters, such as energy input, layer thickness, and scan strategy, can help control the microstructure and phase transformations in MAM, ensuring desirable material properties and performance.
Additionally, post-processing techniques, such as heat treatment, HIP, or stress-relief annealing can be employed to modify the microstructure and promote favorable phase transformations. These techniques can be used to improve mechanical properties, relieve residual stresses, and reduce anisotropy or porosity.
Industry-Specific Material Use & Applications
Metal additive manufacturing has found its way into various industries, enabling the creation of complex, high-performance parts. Here, we explore industry-specific material use and applications for MAM, focusing on aerospace, medical, automotive, consumer products/electronics, and defense
Metal Additive Manufacturing Applications in Aerospace
In the aerospace industry, materials such as titanium alloys, nickel-based superalloys, and aluminum alloys are frequently used due to their high strength-to-weight ratios, corrosion resistance, and excellent high-temperature performance.
MAM enables the production of lightweight, complex geometries and topology-optimized components that help reduce fuel consumption and increase overall efficiency. Typical aerospace applications include engine components, structural parts, fuel nozzles, and heat exchangers.
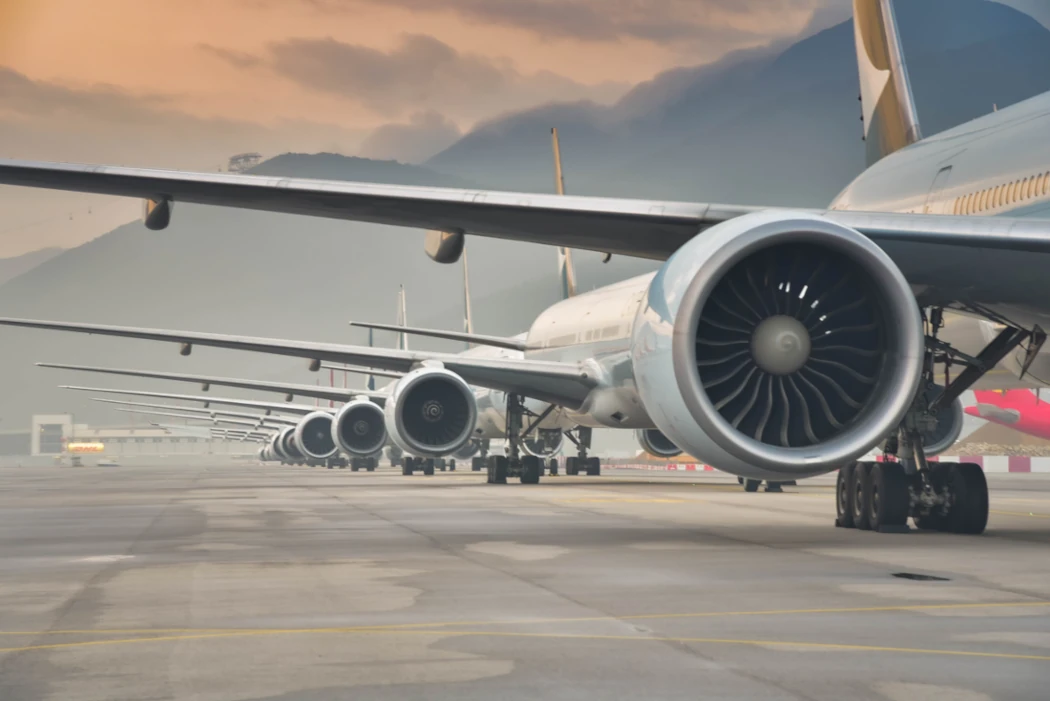
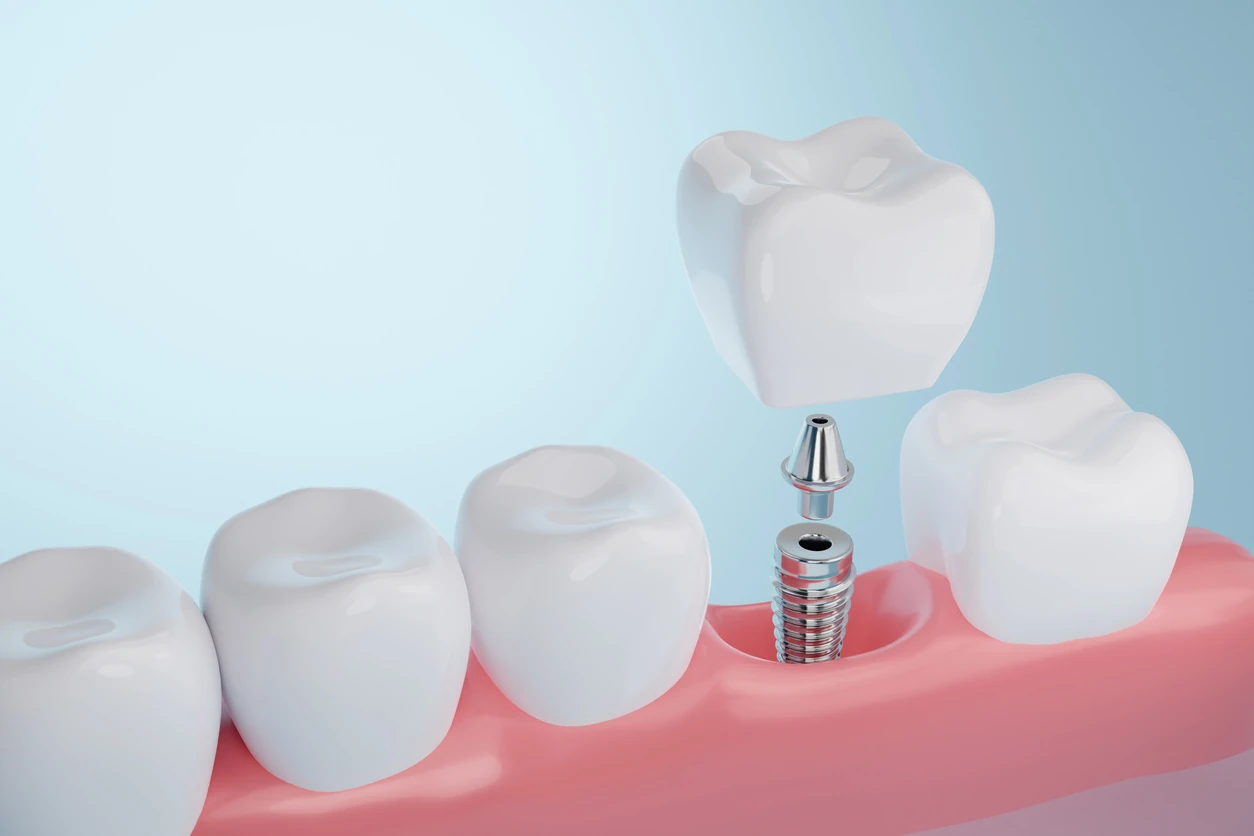
Metal Additive Manufacturing Applications in Medical
Biocompatible materials, such as titanium alloys, stainless steel, and cobalt-chrome alloys, are widely used in the medical industry for their excellent mechanical properties, corrosion resistance, and compatibility with human tissue.
MAM allows for the production of patient-specific implants, surgical instruments, and medical devices with intricate structures and features. Examples of medical applications include orthopedic implants, dental prostheses, and cranial plates.
Metal Additive Manufacturing Applications in Automotive
In the automotive industry, materials such as aluminum alloys, stainless steel, and titanium alloys are commonly used for their lightweight, high-strength, and corrosion-resistance properties.
MAM enables the production of high-performance, lightweight components, and complex geometries that can improve fuel efficiency, reduce emissions, and enhance overall performance. Typical automotive applications include engine components, cooling systems, and suspension parts.
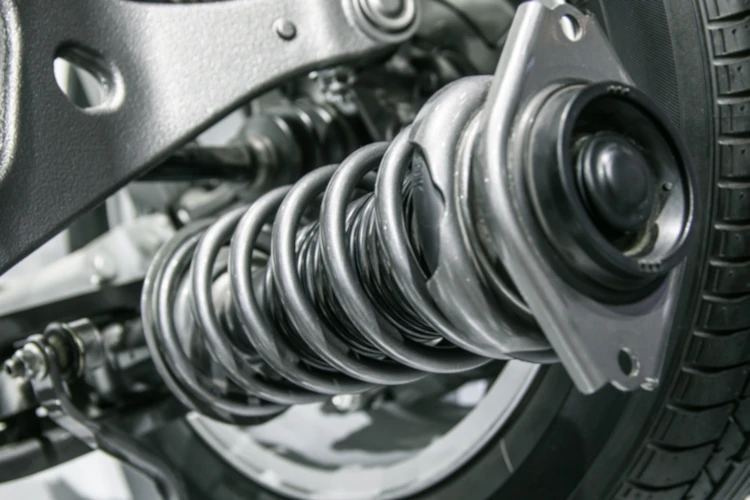
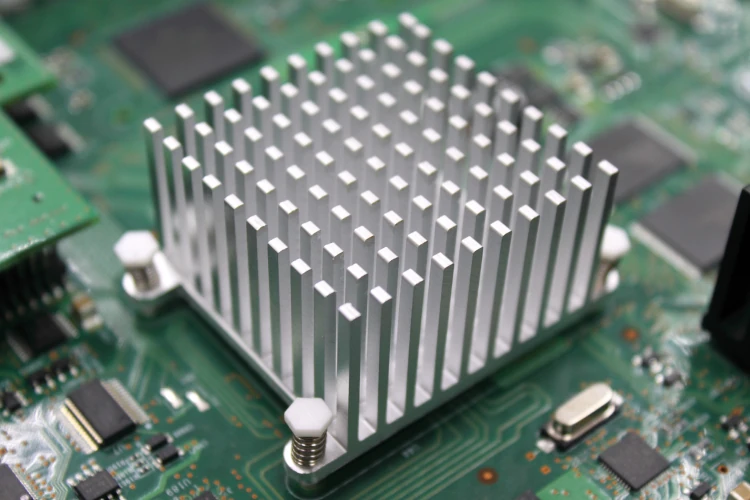
Metal Additive Manufacturing Applications in Consumer Products/Electronics
Materials such as aluminum alloys, stainless steel, and precious metals are often used in the consumer products and electronics industries for their aesthetic appeal, lightweight properties, and corrosion resistance.
MAM allows for the production of intricate, customizable designs and components that can improve product functionality and aesthetics. Examples of consumer products/electronics applications include heat sinks, custom-designed jewelry pieces, high-end watch components, and electrical contacts in electronic devices.
Metal Additive Manufacturing Applications in Defense
In the defense industry, materials such as titanium alloys, stainless steel, and aluminum alloys are commonly used for their high strength, lightweight properties, and corrosion resistance.
MAM enables the production of lightweight, durable, and complex components for various defense applications, including weapon components, unmanned aerial vehicle (UAV) parts, and communication devices.
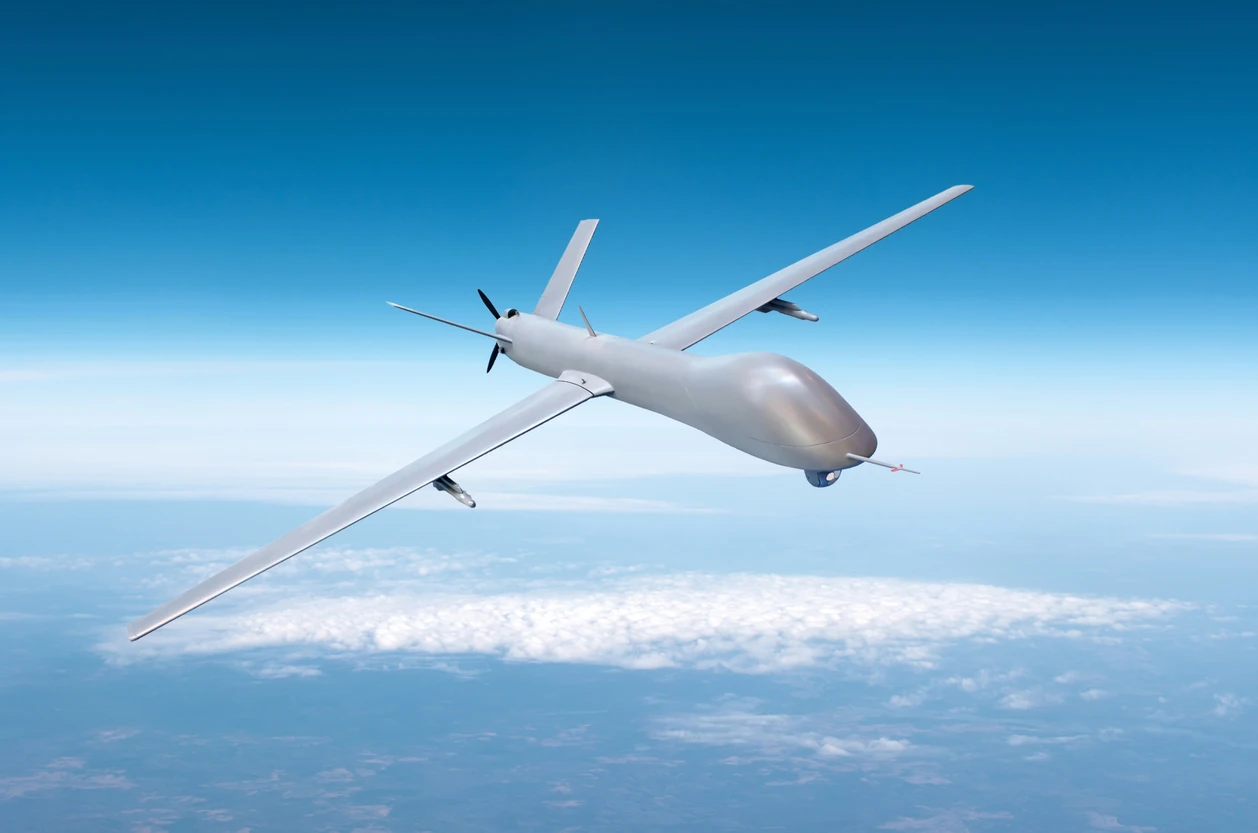
Unlocking the Potential of MAM
The selection of proper materials is crucial for the success of any MAM project. By understanding the unique properties, characteristics, and applications of various metal materials, as well as the factors that influence their performance, you can make informed decisions that optimize part quality, efficiency, and overall production costs.
By leveraging the insights presented here, you can harness the full potential of metal additive manufacturing to create innovative, high-performance components tailored to their specific needs and requirements.
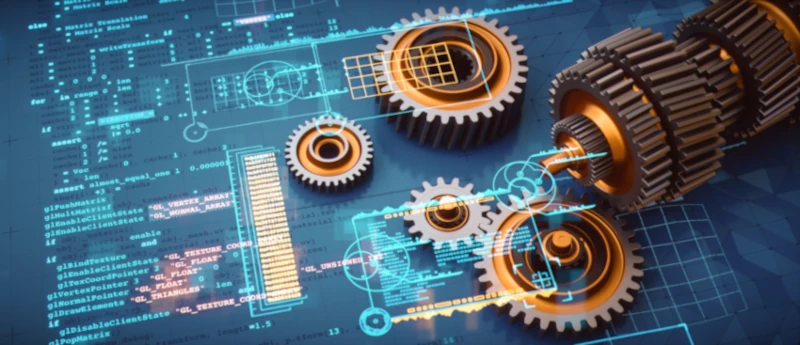